Microwave photonics (MWP) has unlocked a new paradigm for Radio Frequency (RF) signal processing by harnessing the inherent broadband and tunable nature of photonic components. Despite numerous efforts made to implement integrated MWP filters, a key RF processing functionality, it remains a long-standing challenge to achieve a fully integrated photonic circuit that can merge the megahertz-level spectral resolution required for RF applications with key electro-optic components. Here, we overcome this challenge by introducing a compact 5 mm × 5 mm chip-scale MWP filter with active E-O components, demonstrating 37 MHz spectral resolution. We achieved this device by heterogeneously integrating chalcogenide waveguides, which provide Brillouin gain, in a complementary metal-oxide-semiconductor (CMOS) foundry-manufactured silicon photonic chip containing integrated modulators and photodetectors. This work paves the way towards a new generation of compact, high-resolution RF photonic filters with wideband frequency tunability demanded by future applications, such as air and spaceborne RF communication payloads.
Introduction
Radio-frequency (RF) filters play a critical role in maintaining signal fidelity of RF and microwave systems by eliminating unwanted signals and preserving desired signals of interest1. RF filters require fine spectral resolution to operate within crowded RF spectral environments and must also exhibit broadband frequency tunability as operating frequencies of RF systems move toward millimetre wave bands above 30 GHz2. At the same time, they must also achieve small size, weight, and power (SWaP) to meet the demands for operation in future RF applications. However, state-of-the-art RF filter technology fails to simultaneously meet these requirements3. Common RF filter implementations based on surface acoustic waves (SAW) are compact, but face challenges operating at high frequencies. For example, SAW filters have large losses above 3.5 GHz4,5, whereas filters based on bulk acoustic waves can operate into the X-band (8–12 GHz), yet they face fabrication challenges to extend into millimetre wave bands5,6. Yttrium iron garnet (YIG) filters are widely tunable, however their form factor is too large for deployment in SWaP-constrained applications7,8. Another approach is to use switched filter banks which offer compact form factors, although their frequency response cannot be continuously tuned9.
Microwave photonic (MWP) filters overcome the frequency tunability limitations of traditional RF filtering technologies by utilising the broadband tunable and reconfigurable nature of optical components. Moreover, with advances in photonic integration, MWP processors that are fully integrated have the potential to offer the compact footprints required for next-generation microwave systems1,2,10,11,12. Although significant progress on integrated MWP filters has been made, they face difficulties in achieving the fine spectral resolution that is indispensable in RF communication and sensing applications1. One of the most common methods to implement on-chip MWP filters is to use integrated optical micro-ring resonators (MRRs), which exhibit compact form factor, as well as reconfigurable amplitude and phase responses1,13. However, the achievable 3-dB bandwidth of MRR-based MWP filters is typically limited to hundreds of MHz due to waveguide losses10,11,14. Although ultra-high-quality factor (Q) MRRs can be fabricated, they are extremely sensitive to input signal power and temperature fluctuations due to thermo-optic effects15,16, which can add challenges in aligning the laser to the MRR resonance. Additionally, the free-spectral range (FSR) of the MRR can limit the frequency operating range of the resultant MWP processor. MWP filters based on optical tapped delay lines offer highly reconfigurable RF responses, yet are limited in spectral resolution to GHz levels as they require complex amplitude and phase control of many optical filter taps17,18,19. For example, Shu et al. demonstrated an MWP filter that generated an optical comb and processed each comb line on a photonic chip, but due to the limited number of optical comb lines, the filter 3-dB bandwidth was limited to 900 MHz20. Similar to MRR-based processors, the periodic response of tapped delay line MWP processors can limit their frequency operating range.
Stimulated Brillouin scattering (SBS) is an optical nonlinearity first introduced in 192221 that has since re-gained interest for use in integrated MWP filters with fine spectral resolution following the first demonstration of SBS in a photonic chip in 201122. SBS is facilitated through a third-order nonlinear acousto-optic interaction2,21,23,24 and exhibits a narrow intrinsic optical resonant linewidth (10’s MHz) due to the long phonon lifetime. In addition to fine spectral resolution, SBS offers several advantages for MWP processing applications as the Brillouin response does not exhibit an FSR, which does not impose any limitations on the operating range of the resultant MWP system1,2. Furthermore, the thermal stability of the Brillouin frequency shift (BFS), ≈ 1 MHz/°C in optical fibres25, can be much greater than the thermal stability of MRRs, for example, a silicon MRR with Q of 107 exhibited a thermal sensitivity of ≈ 12 GHz/°C16. This highlights the advantages of SBS for MWP processing in terms of tunability and stability.
SBS has been generated in a number of chip-scale platforms22,23,26,27,28,29,30 which enable critical chip-scale MWP processing systems, such as high-performance MWP filters30,31,32,33,34,35,36, phase shifters37,38, true time delays39,40, frequency converters41,42, and high spectral purity microwave sources43,44. Silicon photonic platforms, which have the advantage of being compatible with complementary metal-oxide-semiconductor (CMOS) processes and incorporate an extensive passive and active component library, exhibit large Brillouin gain coefficients23, which is highly desirable for Brillouin-based MWP platforms. Despite these advantages, silicon nanowires on oxide lack intrinsic acoustic guidance, consequently requiring suspended or under-etched structures and exhibit nonlinear optical losses that limit the achievable on-chip Brillouin gain at optical telecommunication wavelengths. On the other hand, silicon nitride (Si3N4) has negligible nonlinear loss, but only offers minuscule SBS gain coefficients30,45 due to the modest elasto-optic coefficient that is intrinsic to Si3N445. Conversely, soft As2S3 chalcogenide glasses offer large Brillouin gain coefficients22 and facilitate more than 30 dB of SBS gain in a compact footprint46, owing to the large elasto-optic coefficient47 and simultaneous confinement of acoustic and optical modes22,46,48. However, chalcogenide waveguides can only be used as a standalone element in MWP filter systems with key MWP components, such as electro-optic (E-O) modulators and photodetectors, remaining off-chip. Previously, we introduced a platform that heterogeneously integrated chalcogenide Brillouin circuits with passive silicon waveguides on a CMOS-compatible SOI platform49. However, the passive platform did not take full advantage of integration with silicon photonics because it lacked an E-O interface. To reduce the SWaP of Brillouin-based MWP processors, integrating chalcogenide Brillouin circuits with the required components of an MWP link, including E-O modulators and photodetectors (PDs), is essential23.
In this paper, we heterogeneously integrate As2S3 Brillouin waveguides in a silicon photonic platform, that includes active E-O modulators and photodetectors, to form the basis of a compact MWP processing platform with fine spectral resolution. We demonstrate an integrated MWP notch filter using on-chip silicon devices and Brillouin gain in As2S3 waveguides for fine spectral resolution processing, achieving 51 dB out-of-band rejection with narrow 37 MHz 3-dB bandwidth and tunable notch central frequencies over 15 GHz. Our demonstration paves the way towards a fully integrated Brillouin-based MWP processor with a reduced footprint and advanced performance.
Click here to read more.
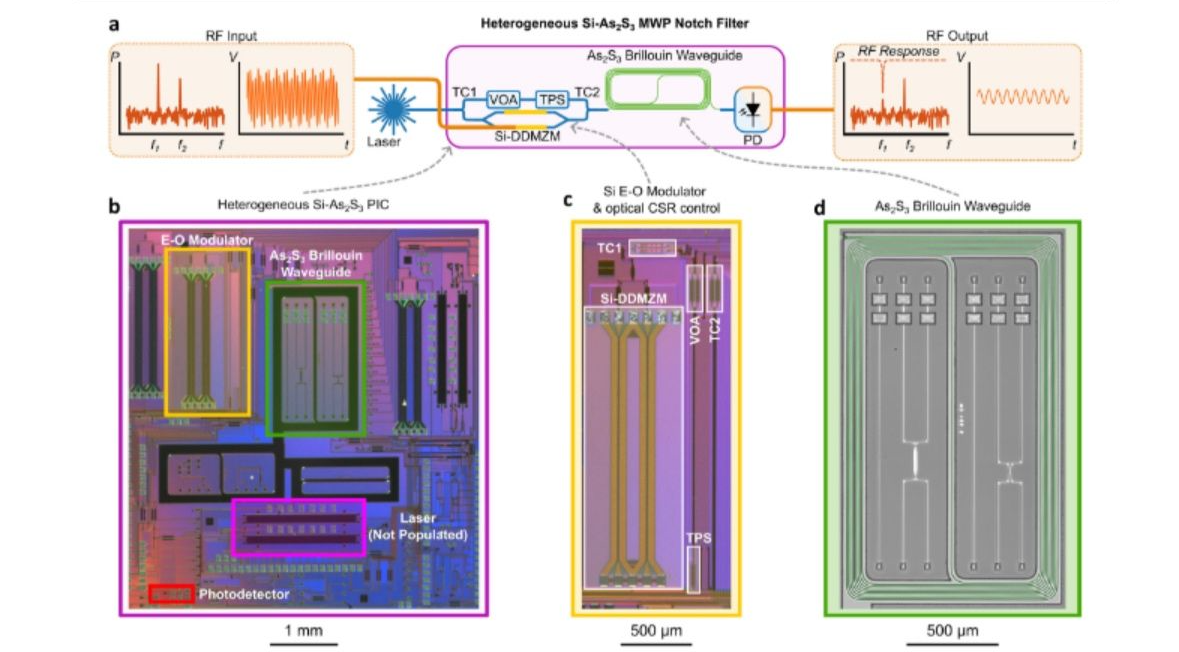